March 17, 2018 9:00 pm
Finding Exoplanets in the Milky Way and Beyond
Today’s guest blog post is by StarTalk intern Kirk Long. Kirk is majoring in astrophysics while minoring in applied mathematics and piano at Boise State University. He spends his summer weekends working at the largest public observatory in Idaho, the Bruneau Sand Dunes State Park Observatory, where he gives educational astronomy presentations and operates various large telescopes for the public. Kirk also helps run the StarTalk Snapchat (startalk-radio).
A brief history of the methods we use to detect exoplanets.
Last month, researchers announced the possible discovery of the first extragalactic exoplanets (and thousands of them!) nearly four billion light years away in an article published in The Astrophysical Journal Letters, a fascinating discovery that relied on some truly mind-bending physics. Authors Xinyu Dai and Eduardo Guerras from the University of Oklahoma used gravitational lensing — a phenomena that Einstein first described as a potential observable effect of relativity, although he never predicted astronomers would actually find any examples of it. This newest discovery (and the fascinating method the researchers utilized) is the latest in a robust tool-kit astronomers have devised to find exoplanets. Since the first exoplanet was discovered in 1992 we’ve discovered more than 3700 of them, and I figured the potential discovery of extragalactic planets was a good time to review the scientific path that brought us to this incredible moment. This list progresses chronologically, so if you’re already an exoplanet expert and want to read more on how gravitational micro-lensing enabled researchers to discover those extragalactic planets, skip to the bottom.
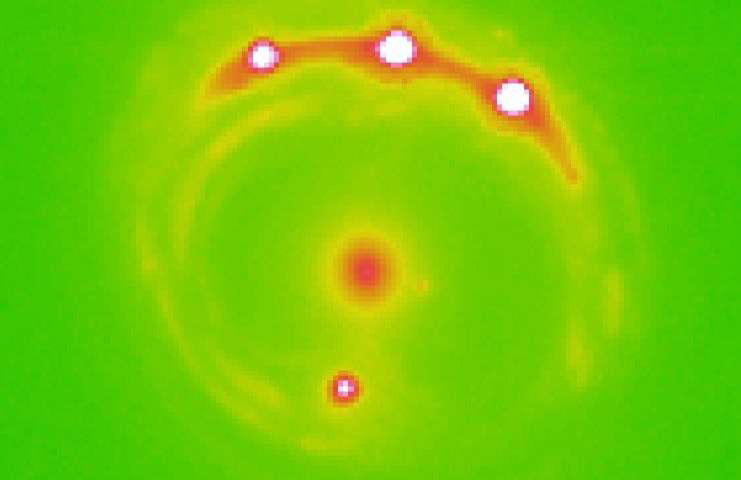
This image (in X-ray) from the University of Oklahoma and NASA’s Chandra observatory shows the dramatic gravitational lensing of the background quasar around the galaxy the researchers studied in their search for the first extragalactic exoplanets. The four bright spots around the edge are all the same quasar, which appears four times as a result of an optical illusion as its light is bent around the foreground galaxy, whose core is visible as the central dot.
1: Using pulsars or variable stars (first confirmed exoplanet — 1992)
The pulsar method was actually the first method that successfully detected an exoplanet, and it’s not used more often for two reasons. First, pulsars — a special kind of fast spinning neutron star formed after a supernova — are relatively rare, and it’s even rarer to find them with planets. Second, pulsars are harsh radiation emitters, and any planets found there would likely not be capable of supporting life as we know it (and one of the main reason we’re looking for extrasolar planets is to potentially find alien life). Despite these pitfalls, the pulsar method is incredibly accurate and works better at relatively longer distances than many of the other methods discussed in this article. It also has a much higher resolution, and is therefore capable of detecting planets even smaller than the Earth at distances pretty far out form the pulsar. A pulsar spins rapidly and consistently, yet if it has a planet attached that timing can be thrown off ever-so slightly as its gravity tugs on it. How significant this wobble in the timing of the pulses we observe here on Earth is allows us to determine a lot about any exoplanets hiding there. A similar approach can be used with other variable stars, but since their timing is often slower and has more variance in it than a pulsar’s it’s less accurate.
2: Doppler shift, or the “wobble” method (First confirmed exoplanet — 1996)
While planets are much less massive than their host stars, they do still exert a gravitational influence on their parents. This causes the star to wobble slightly as the planet orbits it, and there are two methods astronomers use that can detect this wobble. The first (and more common) is the radial velocity method, which utilizes the Doppler effect to determine if a planet is present. Waves can be compressed or expanded depending on how the source is moving relative to the observer, and while you’re probably more familiar with the compression of sound (picture how the pitch of sound changes as a car goes by) light waves can also be compressed. Everything is moving relative to the Earth, and if we analyze a star’s spectra and measure its redshift (how much the light waves have compressed or expanded) we can determine how fast it’s moving away. As the planet is moving around the star, however, it will tug the star in different directions, causing that apparent velocity to change as we observe it from here on Earth, and also causing distinct changes in the redshift of the spectrum. By measuring this tug we can determine an estimate for the mass of the planet, but we can’t really determine much else. This method obviously is easier to see in stars that have lower mass and with planets that have a higher mass (as the tug will be more pronounced) and it also generally requires that the star studied be pretty close to home (so that the spectrum is relatively interference free). One of the oldest ideas for detecting exoplanets, called astrometry, was actually a precursor to the modern radial velocity method. Astronomers as far back as William Herschel (1738-1822) claimed to see the same star “wobble” over time, and deduced that this must be because another hidden object was acting on it gravitationally. While the idea behind this is sound, as astronomy advanced we realized that any such wobble would be so small that it would not be able to be made out by telescopes on Earth, and what Herschel and other astronomers likely saw was our dynamic atmosphere’s interference, causing the “wobble” and not some hidden planet. While the radial velocity method is easier to use (because the differences in spectra are easier to analyze) we have used the physical wobble to find a few exoplanets with space-based telescopes, so it’s not totally impractical.
3: Transit method (first confirmed exoplanet — 1999)
This is the method that has yielded the largest number of exoplanets and has grown the field exponentially. It’s been utilized most famously by the Kepler mission, and NASA’s upcoming TESS mission plans to use this as well. The concept is actually relatively simple — astronomers watch the light coming from a star, and when a planet moves in front of the star it absorbs some of that light and thus there is a corresponding dip in the light we receive here on Earth. It’s an idea that’s been around for a while, but because stars are so bright only relatively recently have we had the instrumentation precise enough to detect it. This method is easy to reproduce and can tell us a lot about a potential exoplanet without us ever directly imaging it, and as our instrumentation and methodology has grown more precise we’ve even been able to start analyzing the atmospheres of potential exoplanets by isolating the planet’s spectrum from the star’s. While it’s certainly a powerful and prevalent tool, everything has its limitations. First, this method can only detect exoplanets if their solar systems are practically edge-on when viewed by us. Second, this method relies on observing a number of transits or other follow-up observations to confirm it is actually a planet (and not just some fluke that happened to dim the star’s brightness momentarily), which means this method can often produce false positives. In addition, in order to observe these transits in a reasonably small amount of time many of the planets must have short orbital periods. Imagine aliens using this method to explore the planets in our own solar system — if they wanted to observe even just two similar transits of every object in our solar system they would have to watch the Sun continuously for two years to discover Earth, and more than 300 years to find Neptune. That’s obviously impractical for our lifespans. You might remember the TRAPPIST-1 system, which was discovered using this method and announced by NASA about a year ago. It made waves in the media because there were seven “Earth-sized” planets in the system, but it really wasn’t that similar to ours. The outermost planet in the TRAPPIST system has an orbital period of just under 20 days, and the whole system would fit inside of the orbit of Mercury if we juxtaposed our solar system on top of it, making it closer in scale to the Jovian system than our solar system. This method is also often used in combination with the radial velocity method to verify exoplanet candidates. While the transit method can determine the radius of a planet and also estimate its distance from the star it can’t directly infer the mass, so adding in the radial velocity method allows us to add in that missing component and gain valuable insight into the physical properties of the exoplanet — density, for example, tells us whether a planet might be rocky (like Earth) or gaseous (like Jupiter), but you need both the mass and the volume to figure that out.
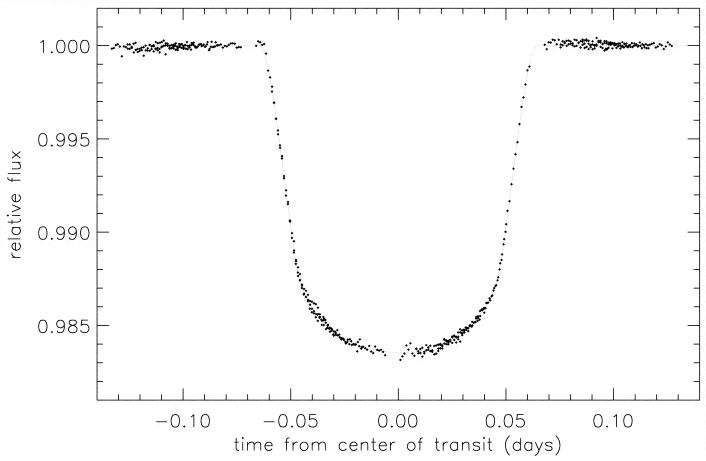
This is a real chart put together from Hubble data that shows the dip in the light curve of a star about 150 light years away. Notice that the dip in starlight is only about 1.5%, and this is for a planet about 1.3 times the size of Jupiter that orbits very close to its host star. For an Earth-sized planet orbiting a sun-like star you’d see an apparent dimming of less than 0.01%. You can find the paper on this hot Jupiter as well as this chart and its supporting data by clicking on the image.
4: Direct observation (first confirmed exoplanet — 2004)
This is perhaps the least-used method on this list, because it requires either a very large telescope, a very large planet, a very small star, a very distant planet, a really close system, a really hot planet, or more realistically some combination of most of those variables. This method also works best when we’re looking at the extrasolar system from directly “above” or “below” it, as the planets will appear to be farthest from the star and thus easier to spot. Planets found this way are usually large and hot, which makes them easier to see in the infrared. Telescopes apply a filter that essentially creates an artificial eclipse, blocking out all the starlight coming from the system and leaving behind just the light emitted/reflected by the planets. The resolution on this method is pretty low with our current technology, and it actually doesn’t tell you all that much about the planets. That, combined with the fact that it’s exceedingly difficult to get the conditions right to accomplish it, means it’s not used as much as some of these other methods.
![Infrared ESO image showing first confirmed exoplanet discovered through direct observation. Credit: The original uploader was Ascánder at Spanish Wikipedia [CC BY 4.0 (http://creativecommons.org/licenses/by/4.0)], via Wikimedia Commons.](https://startalkmedia.com/wp-content/uploads/2018/03/first-exoplanet-direct-image_Credit_Ascánder_Spanish-Wikipedia.jpg)
This image in infrared from ESO shows the first confirmed exoplanet [link: https://en.wikipedia.org/wiki/2M1207b] (left) discovered through direct observation. It’s about 1.5 times the size of Jupiter and located orbiting a brown dwarf about 170 light years away. Credit: By The original uploader was Ascánder at Spanish Wikipedia [CC BY 4.0 (http://creativecommons.org/licenses/by/4.0)], via Wikimedia Commons.
5: Gravitational microlensing (first confirmed exoplanet — 2004)
This is the method used by the researchers who discovered those extragalactic exoplanets mentioned at the beginning of the article, but this method was first applied within our own Milky Way. Einstein proposed that gravity bends the path of light by warping the spacetime it travels through, so massive objects would create interesting illusions if they were between us and a more distant object. While the most pronounced effects are observed around exceedingly massive objects (like galaxies or black holes), anything with mass will bend light to a small degree, even just a star or a planet. The problem with this method is that it requires a precise and rare alignment of stars in space as seen from Earth, and that since everything is in motion that alignment doesn’t last for very long, which makes results obtained hard to verify or repeat. When two stars at different distances line up nearly perfectly, the light from the background star will be bent by the foreground star, causing a distortion of the image we see here on Earth. If the star in front has a planet, that planet’s mass will also bend the path of the light and add to the distortion which can be measured. This produces noticeable spikes in the intensity of the spectrum as the strength of the lense increases as the foreground and background systems appear to move past each other — one small spike as the system passes the planet and another as the system passes the star. Based on the strength of the spectral spike and the timing between them we can approximate the planet’s mass and the distance it is from its host star. Unlike other methods, gravitational microlensing works well on stars like our own, can detect planets far away, and actually works best if the planets aren’t exceptionally close to the host star (otherwise the spikes can be too close together), but it unfortunately is a short-lived and rare occurrence that makes discoveries harder to verify.
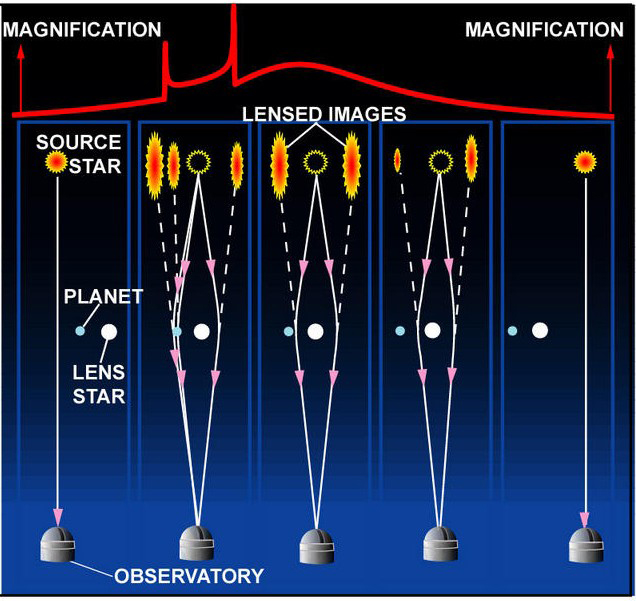
This graphic from OGLE (Optical Gravitational Lensing Experiment) shows the usual process of how a planet might be detected using gravitational microlensing. Credit: OGLE/University of Warsaw.
So what did the researchers do differently to discover exoplanets in another galaxy? In this case, the researchers used a galaxy that’s in front of a quasar — a special and terrifying version of a supermassive black hole that violently expels jets of gas from its poles — causing the quasar’s light to bend around it. By analyzing the spectral shift of light coming from very near the event horizon of the supermassive black hole, the researchers determined that the shifting power of star systems within the lensing galaxy alone was not enough to account for the shifts in certain parts of the spectrum that were actually observed. When they added in a new model for how potential “rogue” exoplanets would additionally alter and lense the light source, their new model fit the observations much better. The researchers estimate that, if these exoplanets were between the mass of the moon and Jupiter, there would be about 2,000 of them for every main sequence star, meaning the potential number of exoplanets found by the researchers could be more than all exoplanets previously discovered (although these rogue planets can’t be individually detected). This may seem like a crazy high number, but even within our own galaxy some astronomers have estimated there could be as many as 100,000 rogue planets for every main sequence star, so 2,000 planets per main sequence star is actually a pretty reasonable number (if you accept that many galaxies are similar to the Milky Way).
But why did the authors of this new study find rogue planets instead of planets orbiting stars? This technique uses tiny variations in the gravitational lensing effect to determine what’s there, and on the galactic scale whether a star system has planets or not doesn’t really change its mass, and since the planets are so close to their host stars (relative to us) it doesn’t really affect the microlensing effect. Rogue planets, while having an even smaller effect than stars, still have an independent effect (since they’re on their own) and it’s that fact that allowed the researchers to model their collective influence on the quasar’s light. With our current technology there’s not really any other way for researchers to verify their findings (aside from repeating the experiment with gravitational micro-lensing) so we may never know for sure whether the planets are actually there or not, but their technique and their model is certainly well thought out. Einstein would surely be amazed to find an effect of relativity that he viewed as unlikely and esoteric being used to discover planets across the universe, and I’m incredibly excited for whatever innovative and surprising ideas researchers will come up with next.
Get the most out of StarTalk!
Ad-Free Audio Downloads
Priority Cosmic Queries
Patreon Exclusive AMAs
Signed Books from Neil
Live Streams with Neil
Learn the Meaning of Life
...and much more